Entry, descent and landing (EDL) is one of the most technically challenging phases of any Mars mission. The low-density atmosphere introduces a number of complications in parachute design, the most important of which is the reduced drag force. To date, all missions achieving successful soft landings on Mars have relied on a single DGB parachute as the primary atmospheric decelerator, based on NASA’s Viking programme. A two-parachute system has been previously investigated: one was used on the Beagle2 Mars Lander in 2003, and one was considered within the ExoMars 2016 program before being rejected in favour of a single disk-gap-band (DGB) parachute based on Huygens heritage. A two-parachute system will be used on the ExoMars RSP mission.
A combined supersonic-subsonic two-parachute system offers potentially significant advantages in meeting the unique challenge of a soft Mars landing. In this system, a first stage supersonic drogue would provide initial deceleration, while a larger second stage subsonic parachute would act as the main decelerating mechanism. This initial drogue would allow the main subsonic parachute to inflate with reduced forces, giving more favourable design parameters. The extensive development history of subsonic Earth parachutes could also be used to inform design, and if a higher drag coefficient could be obtained, an increased landing mass/altitude would be possible.
In order to capitalise on these potential advantages, a subsonic parachute with a high drag coefficient must be designed and characterised. A full understanding of the inflation performance of subsonic parachutes—and their static and dynamic aerodynamic coefficients—is necessary to ensure that they will perform as intended on Mars. The objective of this programme was to develop that understanding.
The objectives of this ESA programme were:
- To identify a suitable parachute for subsonic deceleration on Mars;
- To improve the characterisation of subsonic parachutes used for space exploration; and
- To identify novel test and analysis techniques applicable to parachute research.
Vorticity was the prime contractor for this ESA study which aims at an improved understanding of parachutes operating in the subsonic regime.
In order to achieve the programme objectives, a number of potentially suitable parachute types were selected, and an extensive range of tests were performed. Previous missions, existing parachute designs and available test methodologies were all studied in order to inform the subsonic parachute selection. In addition, a novel parachute geometry—which aimed to improve on the inflation characteristics of the DGB—was designed by Vorticity and included in the test campaign.
In order to build a database of aerodynamic coefficients, and fully describe the inflation profile of the chosen parachutes, four kinds of test (and appropriate subsequent analyses) were performed:
- Static incidence wind tunnel tests (conducted at CNRC, Canada).
- Free-flight deployment wind tunnel tests (conducted at CNRC, Canada).
- Subscale, high-altitude drop tests (conducted at SSC, Sweden).
- Full-scale, low-altitude drop tests (conducted in the UK).
Computational Fluid Dynamics combined with Fluid-Structure Interaction (CFD/FSI) techniques were used to rebuild a number of the tests and to extrapolate the tests to untestable conditions. The results demonstrated the ability to model parachute performance throughout a wide range of operating conditions. Parachute inflation was also modelled successfully. To complement the programme, a number of novel techniques for parachute testing were investigated: the state-of-the-art has been developed considerably for both particle image velocimetry and pressure sensitive paint methods by CNRC.
When combined, these varied methods allowed inflation profiles and extensive aerodynamic databases to be produced successfully for a wide range of subsonic parachutes. In addition, significant practical experience was gained in conducting a complete range of parachute tests, which will be used to inform future work.
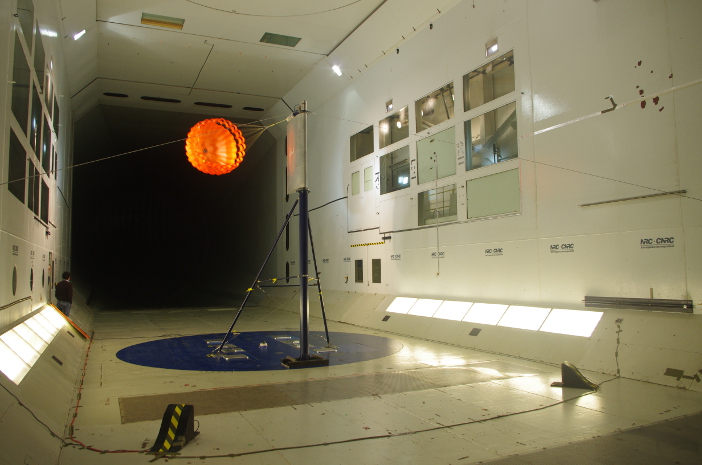
The wind tunnel tests were performed in the National Research Council Canada (NRC) 9×9 m wind tunnel in Ottawa. Two types of tests were performed: firstly, inflation tests where the test parachute was deployed using a drogue, inflated and allowed to fly unconstrained while the drag coefficient was determined; and secondly, coefficient measurement tests where the parachute was constrained at both the line confluence and vent and forced to fly at angles of attack between angles up to ±45° depending on the parachute type while the forces on both constraints were measured. Seven types of model parachute were flown in this test series (2 types of DGB, 2 types of cruciform, 2 novel designs and a tri-conical). All had a nominal diameter of between 1.5 and 2.5 m. The results from the two test series allowed the inflation characteristics and aerodynamic coefficients of the parachute types to be characterised accurately in controlled conditions.
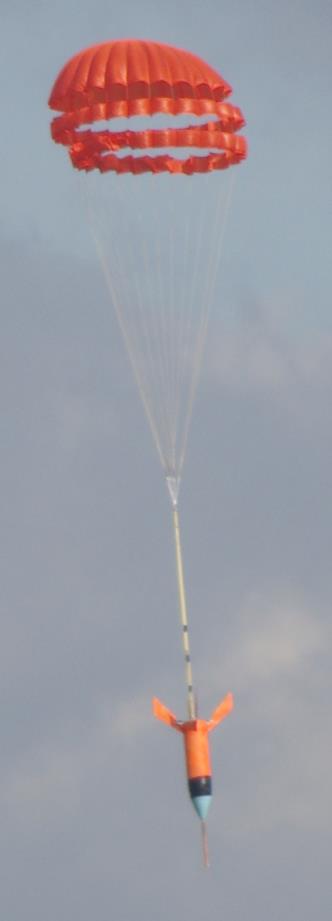
The high altitude drop tests were performed over the SSC test range in northern Sweden using the same model parachutes. In this case, the parachutes were encapsulated in autonomous test vehicles which were lofted to an altitude in excess of 28 km under helium balloons. Once over the test range, the vehicles released themselves from the balloon and accelerated downwards under gravity. On reaching approximately Mach 0.8, a spring drogue was deployed which then extracted the test parachute. The deployment and inflation were recorded using high-speed video and the whole descent using normal speed video. The vehicle motion was recorded using accelerometers, rate gyros and GPS. These tests allowed measurement of the inflation profile under high subsonic conditions, the drag coefficient variation with Mach number and the stability of the system at varying atmospheric density.
The low altitude drop tests were performed in the UK with a Mars-size parachute (8 m diameter) and a 150 kg test vehicle. These were dropped from an altitude of less than 1 km using a helicopter. The test vehicle, which was designed to be easily refurbishable, carried a full sensor suite as for the high altitude drop tests. In this case, a programmer chute was deployed from the test vehicle by static line on release from the helicopter. This controlled the vehicle as it accelerated towards terminal velocity. Once the test condition was reached, the programmer was released, thus deploying the test parachute. Tests were performed with two types of parachute.
In addition to the main test campaigns, two novel techniques for parachute investigation were undertaken: Particle Image Velocimetry (PIV) and Pressure Sensitive Paint (PSP).
PIV allows the flow velocity around an object in a wind tunnel to be measured by seeding the flow with fine droplets of fluid then taking pairs of images a short period apart. These are then analysed to determine the motion of droplets between frames and thus the flow velocity. A 0.75 m DGB parachute was tested in the NRC 2×3 m wind tunnel in Ottawa. The results compared favourably with the results of the CFD/FSI analysis of the same configuration.
PSP is used to determine the surface pressure on objects in wind tunnels; however, it has never been used for flexible structures such as parachutes due to the flexibility requirements for the paint and the low dynamic pressure at which tests with fabrics must take place. Progress was made in formulating paints which were capable of being coated onto fabrics and working at the required dynamic pressure; however, insufficient signal to noise ratio was achieved to allow progress to a wind tunnel test of a full parachute.
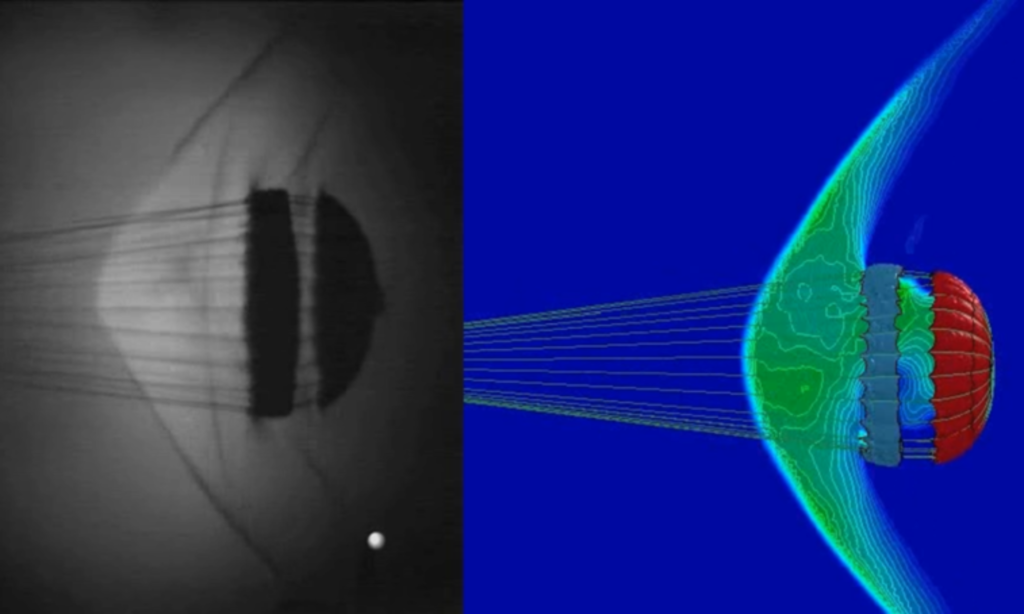
A total of thirteen tests were reconstructed using the CFD/FSI technique. These included wind tunnel tests in the subsonic and supersonic regimes, parachute inflation, PIV tests and free-flight tests. In each case, the motion of the parachute was replicated accurately and the measured force coefficients compared favourably with those measured in the corresponding tests. The flow velocity around the parachute matched the observations from the PIV test accurately. This demonstrated the utility of CFD/FSI to help understand the phenomena observed in tests and to extrapolate test results to untestable conditions.
As a result of this programme the knowledge of subsonic parachutes has been significantly enhanced, a number of test techniques of direct relevance to the development of parachutes for space use have been developed and CFD / FSI has been demonstrated as a valuable analysis tool in this field.
Industrial Team
Vorticity worked throughout the project with the teams from CNRC and Swedish Space Corporation.
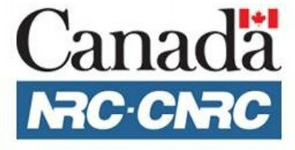
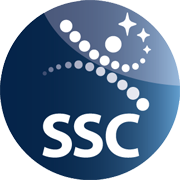